Imagine a world where computers can solve complex problems that are currently impossible for even the most advanced classical machines. What is quantum computing? It is a revolutionary technology trend that holds the potential to transform industries and solve previously unsolvable problems. In this blog post, we will explore the fascinating world of quantum computing, its principles, architecture, applications, and the future it holds.
Table of Contents
Key Takeaways
Quantum computing harnesses the power of quantum mechanics to enable exponentially faster problem-solving capabilities than classical computers.
Quantum processors, gates and circuits manipulate qubits for calculations while key phenomena such as superposition and entanglement are essential for exploiting its potential.
Organizations must invest in research to prepare for the quantum era and take advantage of opportunities offered by this revolutionary technology.
Understanding Quantum Computing
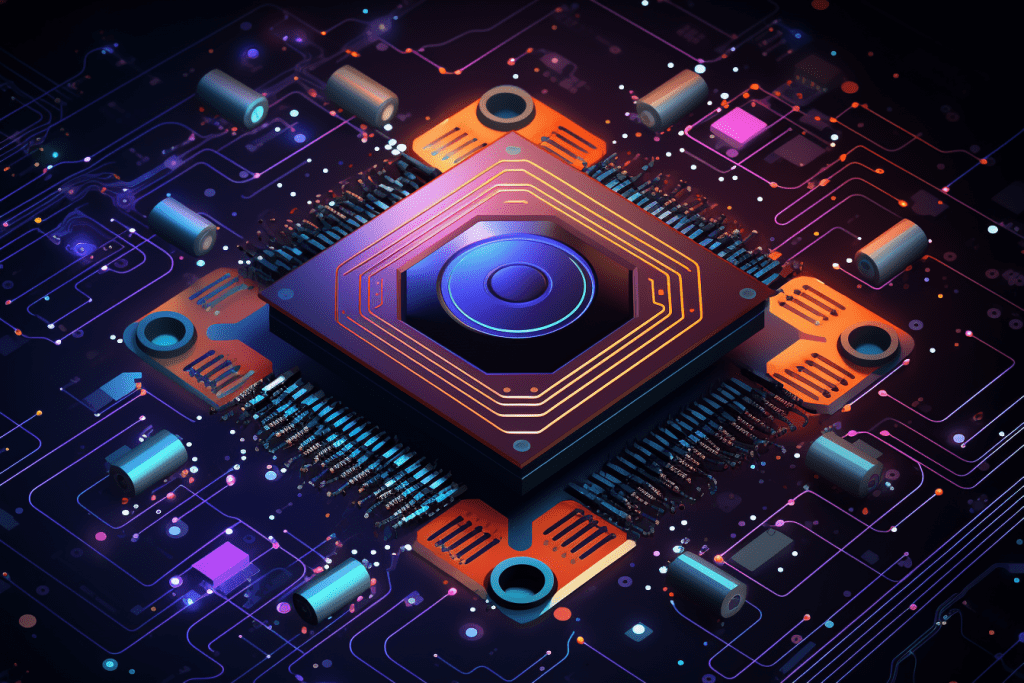
Classical computers use bits that are either zero or one, operating on the principles of classical computing. In contrast, quantum computers operate on the principles of quantum mechanics, using qubits that can exist in both zero and one states simultaneously. This ability allows quantum computers to explore multiple pathways in parallel, providing a quantum advantage over their classical counterparts and the potential to solve complex problems exponentially faster.
The foundation of quantum computing lies in fundamental physics, such as superposition and entanglement, which govern the behavior of quantum particles. Harnessing quantum mechanical effects enables quantum computers to perform calculations that classical machines cannot, leading to advancements in fields like quantum simulation, cryptography, and optimization.
Quantum Mechanics Principles
Quantum mechanics, a fundamental theory in physics, dictates the behavior of particles at the atomic and subatomic scale, serving as the cornerstone of quantum theory. In the realm of quantum computing, principles like superposition and entanglement enable the execution of quantum algorithms that are unattainable using classical computers. These principles, derived from quantum physics, have revolutionized the field.
Superposition, in contrast to classical computer bits that maintain a single state, posits that a quantum particle can simultaneously exist in multiple states. Entanglement, another key quantum phenomenon, allows quantum particles to correlate their measurement outcomes with one another, making it possible to perform intricate calculations that are beyond the capabilities of classical machines.
Qubits vs Classical Bits
Quantum bits, or qubits, the fundamental unit of quantum information, hold a remarkable advantage over classical bits. While classical bits can only maintain a single state, qubits can exist in a superposition of their two “basis” states, allowing for more efficient computation and faster data processing. Additionally, qubits can be entangled, further enhancing their ability to execute complex calculations.
However, qubits also present challenges, as they are highly fragile and prone to errors. Controlling and manipulating qubits is a complex task, making their practical implementation a formidable endeavor. Nonetheless, the potential advantages of qubits over classical bits continue to drive research and development in the field of quantum computing.
The Architecture of Quantum Computers
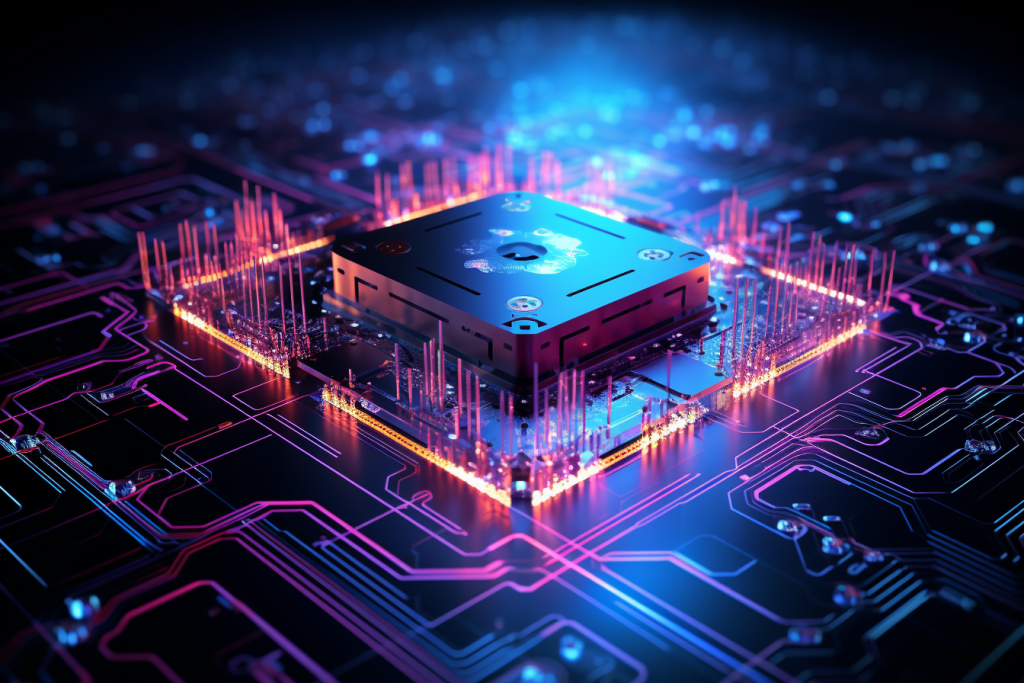
Quantum computers possess a unique architecture, composed of quantum processors and quantum gates, enabling them to perform complex calculations. Quantum processors serve as the building blocks of quantum computers, while quantum gates manipulate the data stored within these processors. This distinctive architecture allows quantum computers to tackle problems that are currently intractable for classical machines.
The potential applications of quantum computing are vast, ranging from quantum simulation to cryptography and security, and optimization and problem-solving. In order to achieve these capabilities, quantum computers must be designed to minimize interference and ensure accurate results.
Quantum Processors
Quantum processors are devices that manipulate qubits to perform calculations. Various technologies, such as ion traps and superconducting circuits, have been developed to build these processors, each with its own advantages and challenges. For instance, superconducting quantum computing uses superconducting electronic circuits to create qubits, offering a promising approach to quantum computing.
Other quantum processor technologies include neutral atom qubit technology, which uses light to trap and maintain qubits at room temperatures, and trapped ion qubits, which are created using atoms from natural sources. Each of these technologies contributes to the ongoing development of more powerful and efficient quantum processors for various applications.
Quantum Gates and Circuits
Quantum gates and circuits play a crucial role in controlling the interactions between qubits and executing quantum algorithms. They manipulate the quantum state of qubits, allowing for the implementation of operations such as quantum simulation, cryptography and security, and optimization and problem-solving.
Understanding the basics of quantum gates and circuits empowers researchers and developers to push forward the field of quantum computing, unlocking its potential to revolutionize numerous industries.
Key Quantum Phenomena
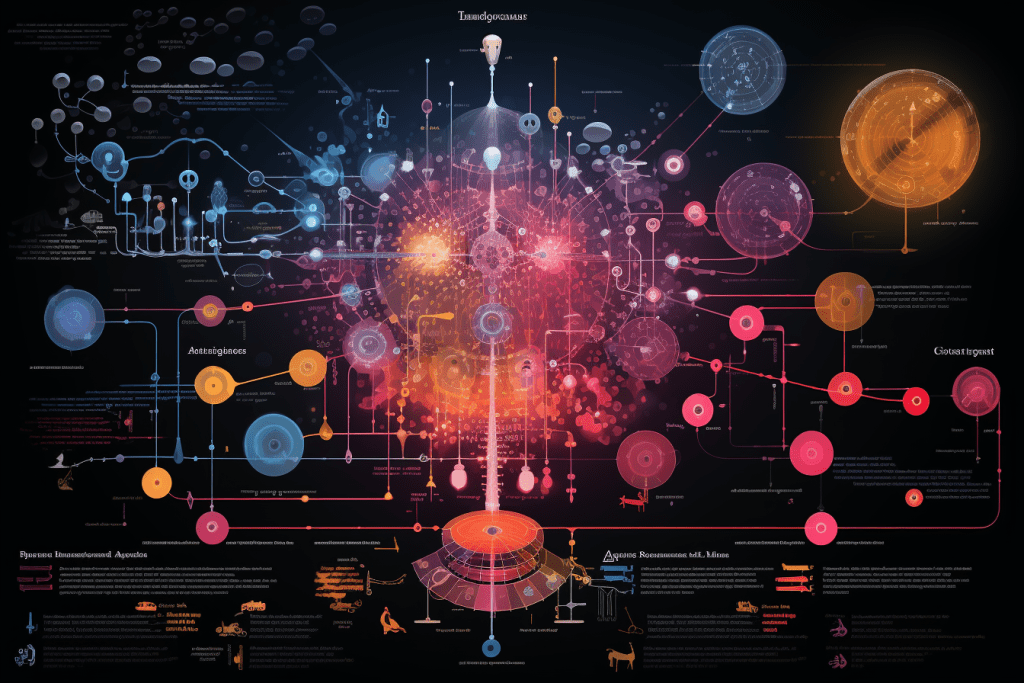
Key quantum phenomena like superposition, entanglement, and quantum interference underpin the success of quantum computing. Superposition allows quantum particles to exist in multiple states simultaneously, enabling quantum computers to explore several paths in parallel and solve problems exponentially faster than classical machines.
Entanglement, another vital quantum phenomenon, allows quantum particles to correlate their measurement outcomes with one another, making it possible to perform complex calculations that are beyond the capabilities of classical machines.
Quantum interference, on the other hand, influences the probability of a quantum system’s collapse as a result of superposition. These phenomena are essential for harnessing the full potential of quantum computing.
Quantum Algorithms and Their Applications
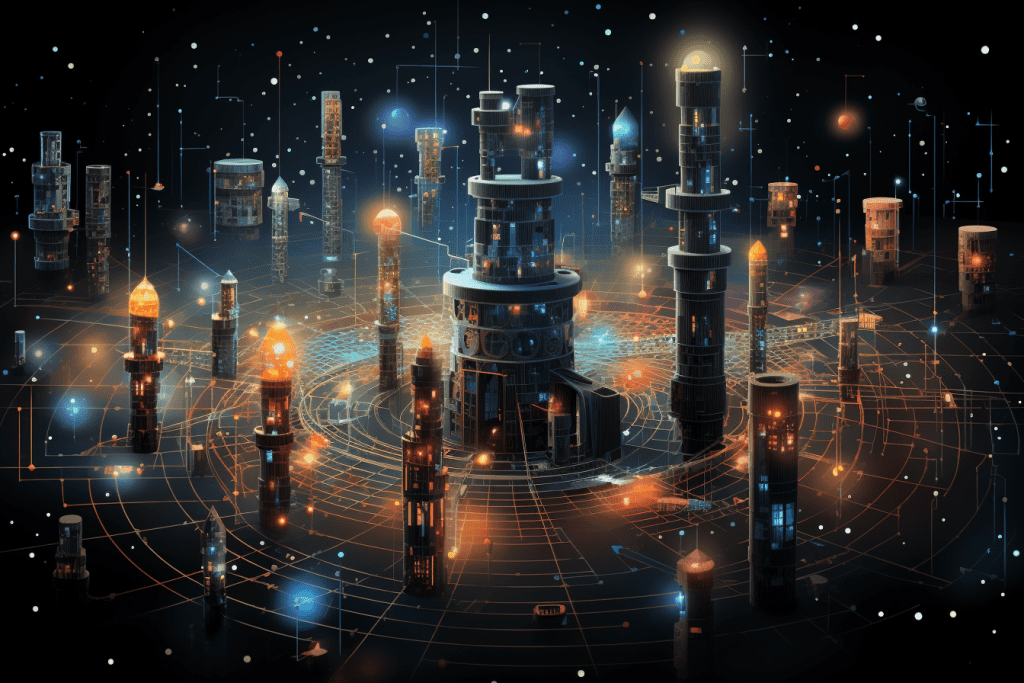
Quantum algorithms, tailor-made for quantum computers, apply quantum mechanics principles to tackle problems beyond the reach of classical computers. One such quantum algorithm has a wide range of applications, including cryptography, search and optimization, and simulation of quantum systems, leading to more efficient problem-solving and breakthroughs in various fields.
Quantum machine learning, for instance, accelerates machine learning processes using quantum software, improving the efficiency of algorithms and enabling new applications in artificial intelligence. As quantum computing continues to advance, we can expect further developments in quantum algorithms and their applications, revolutionizing industries and solving previously unsolvable problems.
Quantum Simulation
Employing quantum simulation for modeling complex quantum systems paves the way for advancements in fields such as materials science and drug discovery. By replicating intricate quantum systems, researchers can gain insights into the behavior of these systems and confront problems that are insoluble on classical computers.
The potential applications of quantum simulation are vast, ranging from molecular simulation for drug discovery to chemical simulations for materials science. As quantum computing technology continues to advance, the potential of quantum simulation will grow, unlocking new possibilities and revolutionizing various industries.
Cryptography and Security
By efficiently solving complex cryptographic problems, quantum cryptography and security offer improved data protection compared to classical computers. Quantum key distribution (QKD), for instance, is a method that utilizes the principles of quantum mechanics to securely transmit data, making it exceedingly difficult for eavesdroppers to intercept or interfere with the information.
As quantum computing continues to develop, we can expect further advancements in cryptography and security, strengthening data protection and enhancing the capabilities of various industries and organizations to safeguard their information.
Optimization and Problem Solving
Quantum optimization algorithms, capable of identifying optimal solutions to intricate problems, enhance decision-making and resource allocation across a range of industries. By executing quantum-inspired optimization algorithms on classical computers, we can uncover solutions that were previously unattainable, leading to more effective management of complex systems such as traffic flows, airplane gate assignments, and package deliveries.
The potential of quantum optimization spans numerous industries and applications, including:
Healthcare
Logistics
Finance
Supply chain management
Drug discovery
Traffic optimization
Portfolio optimization
Machine learning
As quantum computing technology advances, quantum computers could harness the power of quantum optimization, continuing to grow and transforming industries while solving previously insurmountable problems.
Quantum Computing Hardware and Technologies
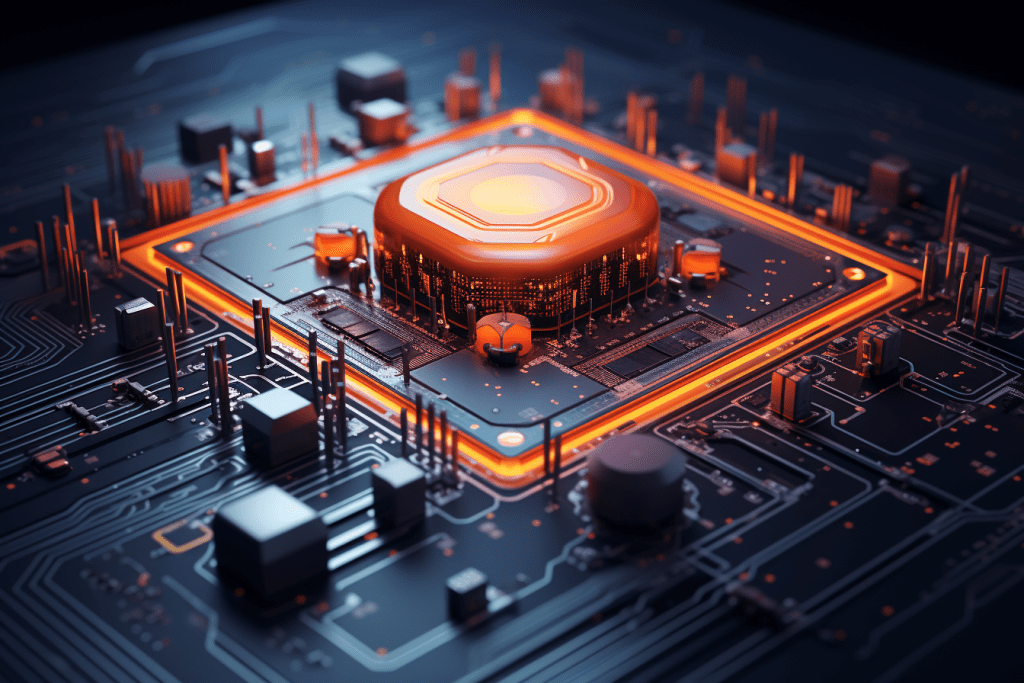
Developments in quantum computing hardware and technologies aim to construct more robust and scalable quantum computers. These components, such as qubits, quantum registers, and quantum reversible gates, are utilized to construct scalable quantum computers capable of resolving intricate problems.
Various technologies, such as superconducting qubits and topological qubits, are being explored to improve the stability and scalability of quantum computers. As research and development in quantum computing hardware continue, we can expect further advancements in the field, leading to more powerful and efficient quantum machines.
Superconducting Qubits
Superconducting qubits, constructed using superconducting electronic circuits, represent a promising avenue in quantum computing. These qubits are comparatively simple to produce and are exceptionally stable, making them a viable option for quantum computing.
Superconducting qubits have several advantages for quantum computing applications:
They possess a long coherence time, allowing them to store quantum information for a more extended duration than other types of qubits.
They are relatively easy to manufacture and control.
They offer stability in their performance.
They have a long coherence time.
These characteristics make superconducting qubits a suitable choice for quantum computing applications.
Topological Qubits and Error Correction
Topological qubits and error correction techniques aim to reduce the impact of quantum interference and improve the stability of quantum computers. Topological qubits, constructed from topological materials, present increased stability compared to superconducting qubits and are less prone to errors.
Error correction techniques involve encoding information in multiple qubits, enabling the detection and correction of errors. By developing topological qubits and advancing error correction methods, researchers can help mitigate the challenges associated with quantum computing and build more stable and reliable quantum machines.
Quantum Computing Challenges and Limitations
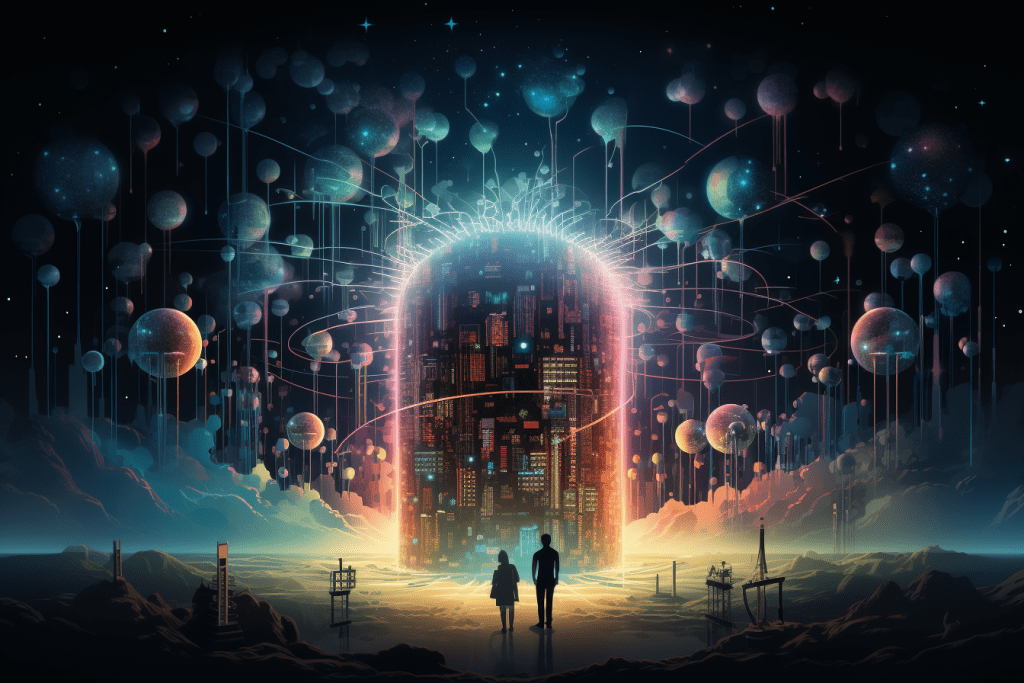
Despite its vast potential, quantum computing grapples with numerous challenges and limitations, including:
Qubit volatility: the tendency for qubits to lose their quantum state due to environmental factors, such as temperature, vibration, and electromagnetic interference
High error rates
The necessity for a myriad of qubits to realize substantial breakthroughs
Error rates, on the other hand, represent the likelihood of a quantum computation producing inaccurate results due to mistakes in the qubits or the quantum circuit.
In order to overcome these challenges and unlock the full potential of quantum computing, researchers must continue to develop advanced hardware, algorithms, and error correction techniques.
The Future of Quantum Computing
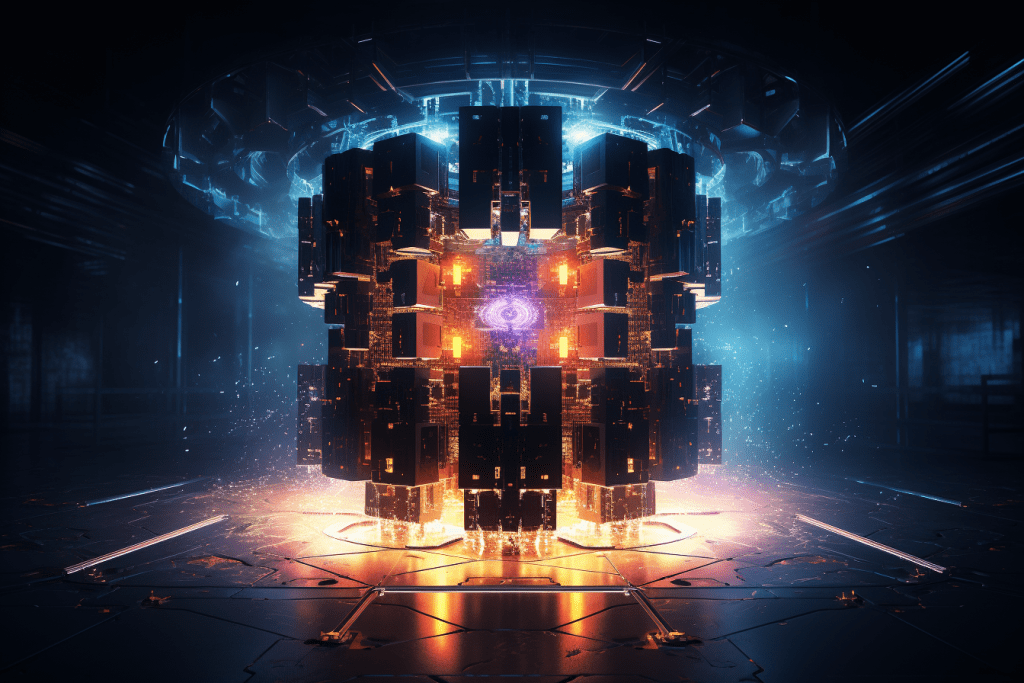
Quantum computing’s future brims with immense potential. Anticipated advancements in hardware, algorithms, and applications are set to transform a variety of industries. As quantum computing hardware continues to develop, we can anticipate more powerful quantum processors, quantum gates and circuits, and improved error correction techniques for quantum computer systems.
Furthermore, the development of quantum algorithms and their applications in quantum simulation, cryptography, and optimization will lead to breakthroughs in areas such as materials science, drug discovery, and secure data transmission. By embracing these advancements and overcoming the challenges and limitations of quantum computing, we can unlock a new era of innovation and problem-solving.
Preparing for the Quantum Era
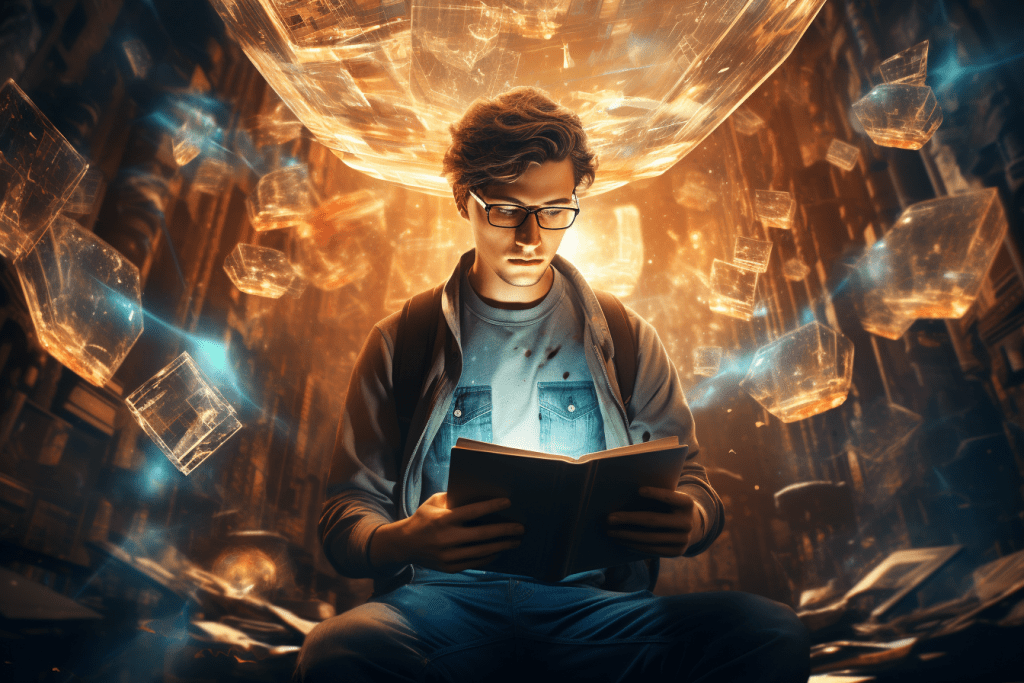
To prepare for the quantum era, it’s necessary to invest in research, cultivate quantum talent, and investigate potential use cases, leveraging the power of quantum computing to maintain a competitive edge. Investment in research will enable businesses and organizations to remain competitive, while cultivating quantum talent can help bridge the talent gap and ensure the successful implementation of quantum computing solutions.
By exploring potential use cases and applications, organizations can capitalize on the value creation opportunities offered by quantum computing and transform their industries.
Embracing the quantum era will require a combination of research, talent development, and practical exploration, ensuring that the full potential of quantum computing is realized.
Summary
Quantum computing is a revolutionary technology that has the potential to transform industries and solve previously unsolvable problems. By leveraging the principles of quantum mechanics, unique architectures, and advanced algorithms, quantum computers can achieve unparalleled computational power and efficiency. As we prepare for the quantum era, investment in research, talent development, and practical exploration is essential to harness the power of quantum computing and revolutionize our world.
Frequently Asked Questions
What is quantum computing in simple terms?
Quantum computing is a new technology that uses quantum mechanics to solve complex problems much faster than classical computers. It combines aspects of computer science, physics, and mathematics to harness the unique behaviors of quantum physics. IBM Quantum is currently making real quantum hardware available to developers.
What does quantum computing actually do?
Quantum computing uses qubits to solve problems that are too complex for traditional computers, harnessing the laws of quantum mechanics to process data exponentially faster than conventional computing. It relies on the superposition of ones and zeros, the digits of the binary system around which all computing revolves, as the basic unit of information. Today, quantum hardware is available to developers worldwide.
Do quantum computers exist now?
Yes, quantum computers exist now and can do some work, with numerous examples existing in scientific laboratories, supercomputer centres, universities, and other places around the world.
What are the key quantum phenomena that underlie quantum computing?
The key quantum phenomena that underpin quantum computing are superposition, entanglement and quantum interference.
What are some potential applications of quantum algorithms?
Quantum algorithms have the potential to revolutionize problem-solving, with applications in cryptography, search and optimization, and quantum system simulation. This could lead to new discoveries and breakthroughs across multiple fields.
Can You Mine Cryptocurrency with a Quantum Computer?
Cryptocurrency mining is a process that involves solving complex mathematical problems to validate transactions and add them to a blockchain. This process is computationally intensive and requires significant processing power. So, how does quantum computing fit into this picture?
Quantum computers, with their exponential computational capabilities, might seem like the perfect machines for mining cryptocurrencies. However, as of now, the practical implementation of quantum computers for cryptocurrency mining is not feasible. There are several reasons for this.
Firstly, quantum computers, as they currently exist, are not yet powerful enough to outperform classical computers in tasks such as cryptocurrency mining. While theoretically, a sufficiently advanced quantum computer could solve the mathematical problems involved in mining incredibly quickly, we are not yet at a point where such a machine exists.
Secondly, the use of quantum computers for mining would require significant advances in quantum algorithms tailored for this process. Currently, these algorithms do not exist.
Lastly, the fragility and instability of qubits, the basic units of quantum information, present a significant challenge. Qubits are highly sensitive to environmental changes, and errors can easily creep into their calculations. These errors could potentially invalidate the mining process, making it unprofitable.
In conclusion, while quantum computers hold great promise for many applications, they are not yet ready to take over the world of cryptocurrency mining. However, as quantum technology continues to advance, this could change in the future.